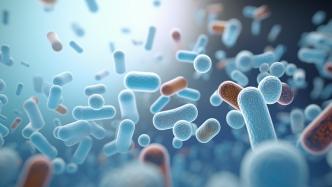
[Editor's Note] With funding from the Shanghai Science and Technology Commission (Project No. 22DZ2304300), The Paper and World Science have conducted popular science reports on the achievements that have won national and Shanghai science and technology awards.
This article focuses on the first prize project of the 2019 Shanghai Natural Science Award "Biosynthesis and Molecular Innovation of Natural Products of Microbial Origin". This award was won by the team of researcher Liu Wen from the Shanghai Institute of Organic Chemistry, Chinese Academy of Sciences.
Gifts of nature - natural products
Active small molecules isolated and extracted from animals, plants, and microorganisms in nature are usually called natural products (NPs). Many of the colored objects we see, the smells we smell, the tastes we taste, and the daily chemicals we use come from natural products. As the selfless original gift of nature, natural products are a huge treasure house, containing secrets that humans have not yet completely uncovered. From the ancients using fermentation to obtain gallic acid from gallnuts, to Li Shizhen using the sublimation method to prepare and purify camphor, to the German pharmacist who first isolated the single compound morphine from opium poppy, humans have been exploring and exploring natural products for thousands of years. The decryption never stops.
There are many star molecules in natural products, such as artemisinin, penicillin, erythromycin, etc. Their discovery and application have made important contributions to human health and disease treatment. The discovery of artemisinin was inspired by traditional Chinese medicine classics and was extracted, identified and synthesized by Chinese scientists from the traditional Chinese medicine Artemisia annua. Artemisinin is widely used in malaria treatment, benefiting hundreds of millions of people around the world. The main discoverer, Professor Tu Youyou, became the first Chinese scientist to win the Nobel Prize in Physiology or Medicine. Penicillin was the first antibiotic discovered. It was accidentally observed by British doctor Alexander Fleming from a moldy bacterial culture medium. He named the chemical produced by the mold penicillin. Penicillin can kill many kinds of bacteria, thus creating the history of modern antibiotics and saving the lives of countless patients. Erythromycin is another important antibiotic discovered after penicillin. It was extracted from soil bacteria in the Philippines by Eli Lilly and Company. Erythromycin can treat a variety of bacterial infections and provides an effective treatment plan for many patients. It is one of the indispensable drugs. Among the above three star molecules, penicillin and erythromycin are both derived from microorganisms. Microorganisms are tiny organisms that exist widely in nature. They have rich metabolic capabilities and can synthesize various complex natural products. These active small molecules derived from microorganisms also play an important role in all aspects of our lives.
Producing natural products of microbial origin in daily life
Agriculture Until now, chemical pesticides have been the first choice for controlling pests in agricultural production. Due to the harm of chemical pesticides to the environment and potential risks to the human body, biological pesticides with strong insecticidal capabilities, environmental friendliness, and low toxic and side effects on the human body seem to be a better choice. Abamectin is a biological pesticide derived from soil bacteria. It can interfere with the neurophysiological activities of pests and quickly paralyze them. Because of its significant insecticidal effect and extremely low dosage, it is very safe for humans, animals and the environment.
How industry produces industrial products in a sustainable, green and clean way is crucial to ensuring sustainable social and economic development. In addition to bulk industrial products such as alcohol, vinegar, and lactic acid produced by microbial fermentation, microorganisms can produce natural dyes with bright colors, such as indigo and carotenoids, which are widely used in the textile and printing industries. Microorganisms are also capable of producing compounds with special aromas and flavors, such as banana ketone and eugenol, which are used as additives in perfumes and flavors.
Why are probiotic foods , which can be seen everywhere in our lives, so popular? Because probiotics can promote the absorption of intestinal nutrients, remove or reduce the adhesion of pathogenic bacteria, maintain the balance of intestinal flora structure, and improve the symptoms of constipation, diarrhea and indigestion. Lactic acid bacteria are a common type of probiotic bacteria that can synthesize lactic acid and other organic acids through fermentation. In addition, lactic acid bacteria also produce some active substances, such as lactobacilli and lactobacilli, which have antibacterial, antioxidant and other biological activities.
Skin care products In terms of skin care, natural products of microbial origin also show amazing advantages. Yeast is a common microorganism in the field of skin care products. Through the fermentation process, it can produce a variety of small molecule compounds, including polypeptides, polysaccharides, polyphenols, etc. These substances can promote skin metabolism and increase collagen synthesis, thereby achieving moisturizing, anti-wrinkle, whitening and other effects.
Natural products derived from medical microorganisms have complex structures and have important applications and prospects for continued development in the field of medicine. Secondary metabolites such as β-lactams, macrolides, and aminoglycosides derived from microorganisms have been widely used. In the medical fields of anti-infection, anti-tumor, immunosuppression, and hypoglycemia. Compared with traditional chemically synthesized molecules, these active small molecules derived from microorganisms often have clear targets and significant drug effects, which bring advantages and challenges to drug discovery and innovation.
Newer and iterative natural products of microbial origin
Although microorganisms in nature are widely distributed, the types and yields of various products they can produce are still limited. Therefore, enriching the types of chemicals produced by microbial cells and improving the production efficiency of cells have become two key issues in the field of research related to microbial-derived natural products.
On the one hand, for the problem of obtaining new varieties of natural products derived from microorganisms, the current main solutions include fully developing microbial resources to find new molecules through genome mining methods, and modifying known natural products through modification of metabolic pathways. Get new molecules. Due to evolutionary characteristics, genes encoding natural products often exist in clusters in the genomes of microorganisms, which makes it easy to mine and transform the natural products they produce. There have been a large number of research reports on the mining of natural products derived from microorganisms. For example, ribosomal peptides have become the main source of active small molecules and are being discovered and characterized at an unprecedented speed and scale, becoming potential stocks for the development of new drugs. Likewise, there are increasing cases of artificial modification of the biosynthetic pathways of natural products to obtain unnatural “natural products”. For example, anidulafungin can be used clinically to treat deep fungal infections. Its production process is based on the small molecule mother core produced by fungal fermentation, and the key structural modifications are achieved through microbial transformation.
On the other hand, many natural products of microbial origin do not meet the requirements for large-scale production. Many active small molecules are often calculated in milligrams, and the yield is very low and not economically feasible, which may directly lead to the inability to produce or the high price of the corresponding products. In response to this problem, early solutions mainly used natural screening and mutation breeding to obtain high-yield microorganisms. However, this irrational strategy is extremely inefficient. With the continuous development of biological technology, advances in the ability to understand and modify microbial systems have led to the birth of "synthetic biology" (a discipline that combines life science observation and analysis methods with engineering design thinking). From the perspective of synthetic biology research, some silent genes that are not originally expressed can be activated in microbial hosts, some genes derived from animal and plant systems can also be expressed, and even artificially synthesized genes that do not exist in nature can be expressed. Gene.
The smallest factory in the world - microbial cell factory
Microorganisms are an important source of drug discovery and development, and are also an ideal "cell factory" for efficient and precise preparation of drugs. When people think of factories, they should think of fixed buildings, mobile workers, and fast logistics; when it comes to microorganisms, you may think of those lurking around us that may cause food spoilage, wound infections, and infectious disease outbreaks. An invisible and intangible little guy. So, how are factories and microorganisms connected? Generally speaking, a factory refers to a production system that is manually designed to have certain production lines, equipped with corresponding power and other auxiliary systems, and operates under certain management procedures. A rationally designed microbial cell is like a "micro factory". After inputting raw materials, the genetic information in its body is like a smart computer that quickly runs the program. Driven by metabolic energy, the designed and optimized "production line" begins to operate. Finally output a specific product. Microbial cell factories have the advantages of high yield, low cost, easy operation, green and environmental protection, and are widely used in biomedicine, biomaterials, food, cosmetics and other fields.
In order to adapt to large-scale industrial applications, microbial cell factories need to be modified. The range of raw materials for an ideal microbial cell factory can be continuously expanded to maximize energy absorption and product yield. As the yield approaches the theoretical maximum, whether it is from the upstream strain construction, midstream amplification effect, or downstream industrial production, it will be more challenging to further increase the yield, which requires more reasonable and systematic engineering. Strategy.
How to make microorganisms, the cell factories, more efficient?
Through in-depth research on the genomics, proteomics, metabolomics, and transcriptomics of microorganisms, we can discover the action mechanisms of many metabolic pathways and enzymes, and build efficient microbial cell factories based on the understanding of these mechanisms. With the update and iteration of biological technology, many genetic engineering, protein engineering, and metabolic engineering strategies for constructing microbial cell factories have been developed, which can help microbial cell factories break through bottlenecks in complex application environments and increase the yield of target products.
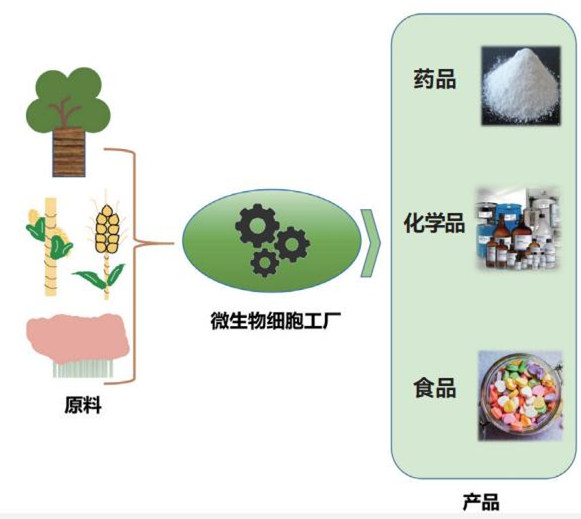
Genetic engineering refers to the use of molecular biology and microbial genetics methods to recombine, clone and express genes according to a pre-designed blueprint (i.e. recombinant DNA technology). It involves the artificial modification of DNA by introducing exogenous genes or Change the expression of its endogenous genes to control and improve specific traits. Protein engineering refers to the use of molecular biology techniques to modify the structure and function of proteins. Through the purposeful design and modification of genes encoding proteins, the properties of proteins are controlled, such as improving their stability, activity, antigenicity, solubility and Immunogenicity, etc., to adapt to different application needs. Metabolic engineering refers to the regulation and optimization of target product yields, metabolic pathways and metabolites by transforming and regulating cellular metabolic networks, so that they can effectively synthesize the required compounds. This usually involves the analysis of microbial genomes, transcriptomes, and proteomes. and analysis and regulation of the metabolome.
At the same time, information analysis as an auxiliary and guidance tool is also advancing with the times, and the development of bioinformatics has pushed microbial research into a new era. Utilizing biological components from the extremely complex and dynamic living environment within cells, a "Design-Build-Test-Learn" (DBTL) iterative cycle was developed that relies on data analysis and mathematical modeling with the goal of functional characterization and response feedback, Construct a more efficient and simple microbial cell factory. There is no doubt that these extensive and in-depth studies will provide great potential for the development of better natural products of microbial origin.
In recent years, synthetic biology has developed rapidly as an emerging discipline. Through synthetic biology technology, the metabolic capacity of microbial cell factories can be optimized, and the biosynthetic process of natural products can also be optimized. In addition, based on the understanding of natural product biosynthesis, synthetic biology technology can be used to transform microbial cell factories to achieve targeted modification of target metabolites and generate unique products that are difficult to obtain in nature.
Biosynthesis and molecular innovation of natural products of microbial origin
The purpose of natural products produced by microorganisms is not for human use, but as tools or media to deal with various external environmental concerns, such as signal transduction, competitive antagonism, and environmental adaptation. These biological processes are often dynamic and variable, putting forward new synthetic requirements for organisms and adapting to changes in various biological functions through structural changes, optimization and final selection of their products. Therefore, the interaction between organisms and their internal and external environments promotes the co-evolution of natural products and biology, and also directs the basic laws of small molecule evolution in nature based on universal and specific laws. Understanding the universal and specific laws of natural product biosynthesis, and artificially accelerating the evolution of small molecules based on these understandings, will greatly promote the discovery, expansion of structural diversity and application of microbial-derived natural products.
Researcher Liu Wen's team at the Shanghai Institute of Organic Chemistry, Chinese Academy of Sciences, follows the research idea of "learning from nature, understanding nature, and innovating from nature" and focuses on the establishment of biosynthetic pathways of natural products and new enzymes in microbial production systems. He is committed to elucidating the scientific mechanism, developing new strategies for natural product discovery, and using the concepts of synthetic biology to accelerate the evolution of small molecules and expand their uses. He has achieved a series of leading results that have been highly praised and widely watched by domestic and foreign peers. The team systematically revealed the biosynthetic mechanisms of multiple representative antibiotic families such as lincosamides, thiopeptides, and spiroacetoacetolactones, which greatly enriched the understanding of natural small molecules based on universality and specificity. The theory of the laws of natural evolution of products. For example, lincosamide antibiotics are a type of sulfur-containing antibiotics produced by Streptomyces. Among them, lincomycin is widely used to treat infections caused by Gram-positive bacteria and has been used clinically for more than half of the time. century. However, the biosynthetic mechanism of this family of antibiotics has not been clear, which seriously hinders the development and efficient manufacturing of related new drugs. Liu Wen's team published an article in Nature, revealing the biosynthetic mechanism of lincomycin involved in two small molecule thiols - ergothioneine (EGT) and actinomycin (MSH) - breaking the This has overturned people's long-term cognitive constraints on the detoxification function of small molecule thiols that are widely present in organisms, and revealed a new way for sulfur to be introduced into natural product molecules . In subsequent studies, the team has successively revealed the important role played by more functional proteins derived from degradation or detoxification pathways in microorganisms in the construction of active molecules lincosamide antibiotics, explaining the unbreakable and unified unity of opposites embodied in microbial biosynthesis. The laws of nature.
Although the structures of natural products derived from microorganisms are complex and diverse, in microorganisms, they all use simple primary metabolite molecules such as small molecule carboxylic acids, amino acids, and monosaccharides as substrates, follow certain chemical principles, and work through the sequential cooperation of a series of enzymes. Formed by catalytic reaction. The discovery of new and unique enzymes in the biosynthesis process of natural products and the analysis of their enzymatic mechanisms will enrich the components of synthetic biology and are the key to successfully using synthetic biology methods to build microbial "cell factories". During the backbone construction process of the spiroacetoacetolactone antibiotic pyrroloindolimycin, the team discovered two different enzymes that can greatly promote the Diels-Alder (DA) cyclization reaction. . The DA reaction is one of the most important reaction types in synthetic chemistry. As an extremely effective means of chiral construction of carbon-carbon bonds, it is widely used in synthetic chemistry, medicinal chemistry, materials chemistry and other fields. Scientists have long predicted the DA reaction. It may exist in biological systems, but for decades it has not been possible to definitively determine whether the DA reaction enzyme exists naturally. The discovery creates an opportunity to answer this long-debated but unsolved scientific puzzle in biology and chemistry. The team discovered a new S-adenosylmethionine (SAM)-dependent enzyme protein that catalyzes free radical-mediated enzymatic structural rearrangements in the thiopeptide antibiotic biosynthetic pathway. There are tens of thousands of free radical enzymatic reactions mediated by SAM in nature, but the relevant enzymatic mechanisms are poorly understood, largely due to a lack of understanding of related products and free radical transformation processes. The discovery of this enzyme and the revelation of its catalytic mechanism are an important step in understanding the complex structural rearrangement reactions catalyzed by SAM-dependent free radical proteins, which have unique structural units and important pharmaceutical activities, especially aromatic structural units. chemical molecules are of great significance.
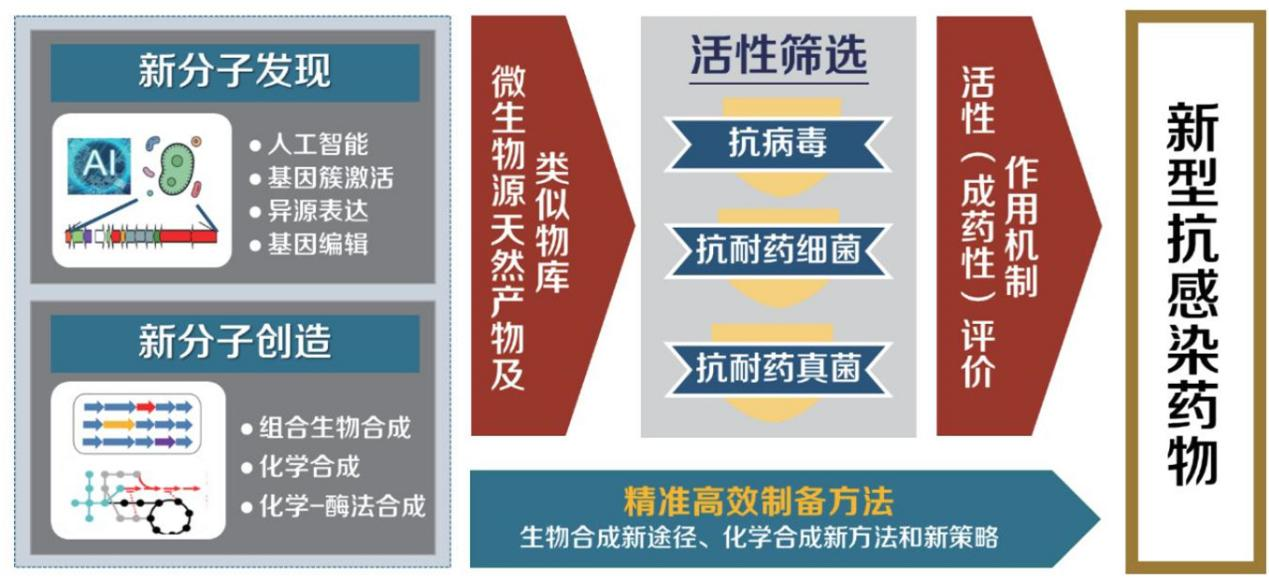
Liu Wen’s team’s research strategy
Based on the principle discovery of the biosynthetic mechanism of natural product molecules, Liu Wen's team actively expanded the application of theoretical research in methodology, used biotechnology to solve problems in synthetic chemistry, and expanded the structural diversity and uses of natural products derived from microorganisms. He proposed the concept of "multiplexed combinatorial biosynthesis", applying the idea of combinatorial chemistry to all stages of biosynthesis to maximize the diversity and uses of molecules. Taking the antimycin-resistant Streptomyces production system as a model, a diversity-oriented biosynthetic strategy was used to construct a dilactone natural product analog library containing hundreds of members; through screening, a large number of bilactone natural product analogues with higher activity than the parent were obtained. Structural monomers of compounds and introduce a large number of pharmaceutically important, chemically active or naturally non-existent functional groups. Based on the analysis of the systemic commonality and individual biosynthetic mechanisms of thiopeptide antibiotics, combined with the concept of synthetic biology, dozens of new thiopeptide antibiotics were designed and biosynthesized using bacteria as "cell factories" , among which fluorine Compared with the reference drug and vancomycin, which is used as the last line of defense clinically, the substituted thiostrepton derivatives are more than 200 times more active against drug-resistant pathogenic bacteria. They are excellent anti-infective drug leads and inspire This sparked an international craze for using “cell factories” to derive thipeptide structures. Currently, more new thiopeptides have been created biosynthetically than have been naturally isolated over the past 70 years combined .
In addition, the team pays attention to national needs and is committed to breakthroughs in some key scientific issues in the industrialization of bulk antibiotic products in my country and the application and transformation of corresponding theories and methods. The research team has improved the yield and quality of products by designing new engineered strains of antibiotics such as erythromycin, lincomycin, and abamectin using synthetic biology technology, and uses the concept of synthetic biology to accelerate the evolution and expansion of small molecules. Its use has set a good example in the technological development and transformation of bulk industrial fermentation products.
In the future, the research trend on efficient microbial cell factories is to combine synthetic biology with other disciplines, such as computer science, artificial intelligence, and machine learning, to achieve more precise design and prediction. The combination of these technologies will accelerate the discovery and application of active small molecules and promote the further development of biosynthesis and molecular innovation of microbially derived natural products.
(The author Liu Wen is the deputy director, researcher and doctoral supervisor of the Shanghai Institute of Organic Chemistry, Chinese Academy of Sciences, and the first recipient of the first prize of the Shanghai Technology Invention Award; Zhao Qunfei is a researcher, master's supervisor of Shanghai University of Traditional Chinese Medicine, and the first recipient of the Shanghai Technology Invention Award. The second recipient of the first prize of the Invention Award; Chen Dandan is an associate researcher at the Shanghai Institute of Organic Chemistry, Chinese Academy of Sciences; Yue Houru is a graduate student at the School of Life Science and Technology, Shanghai Jiao Tong University.)